What is Crystal Field Theory?
Crystal field theory describes
the net change in crystal energy resulting from the orientation of d orbitals of a transition metal cation inside a coordinating group of anions also called ligands.
A major feature of transition metals is their tendency to form complexes. A complex may be considered as consisting of a central metal atom or ion surrounded by a number of ligands. The interaction between these ligands with the central metal atom or ion is subject to crystal field theory.
Crystal field theory was established in 1929 and treats the interaction of metal ion and ligand as a purely electrostatic phenomenon where the ligands are considered as point charges in the vicinity of the atomic orbitals of the central atom. Development and extension of crystal field theory taken into account the partly covalent nature of bonds between the ligand and metal atom mainly through the application of molecular orbital theory. Crystal field theory is often termed ligand field theory.
Overview of Crystal Field Theory
In order to understand clearly the crystal field interactions in transition metal complexes, it is necessary to have knowledge of the geometrical or spatial disposition of d orbitals. The d-orbitals are fivefold degenerate in a free gaseous metal ion. If a spherically symmetric field of negative ligand filed charge is imposed on a central metal ion, the d-orbitals will remain degenerate but followed by some changes in the energy of the free ion.
A summary of the interactions is given below.
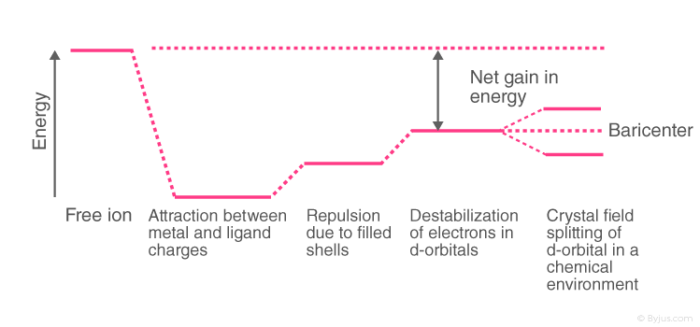
Crystal Field Splitting
Crystal field theory was proposed which described the metal-ligand bond as an ionic bond arising purely from the electrostatic interactions between the metal ions and ligands. Crystal field theory considers anions as point charges and neutral molecules as dipoles.
When transition metals are not bonded to any ligand, their d orbitals degenerate that is they have the same energy. When they start bonding with other ligands, due to different symmetries of the d orbitals and the inductive effect of the ligands on the electrons, the d orbitals split apart and become non-degenerate.
High Spin and Low Spin
The complexion with the greater number of unpaired electrons is known as the high spin complex, the low spin complex contains the lesser number of unpaired electrons. High spin complexes are expected with weak field ligands whereas the crystal field splitting energy is small Δ. The opposite applies to the low spin complexes in which strong field ligands cause maximum pairing of electrons in the set of three t2 atomic orbitals due to large Δo.
- High spin – Maximum number of unpaired electrons.
- Low spin – Minimum number of unpaired electrons.
Example: [Co(CN)6]3- & [CoF6]3-
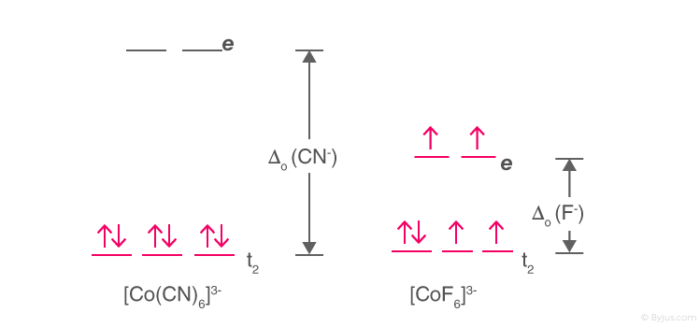
High Spin and Low Spin Complex
- [Co(CN)6]3- – Low spin complex
- [CoF6]3- – High spin complex
Recommended Videos
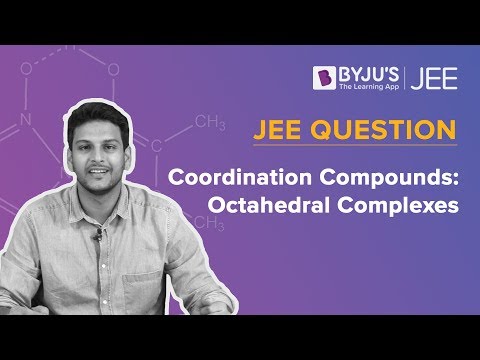
The pattern of the splitting of d orbitals depends on the nature of the crystal field. The splitting in various crystal fields is discussed below:
Crystal Field Splitting in Octahedral Complex
- In the case of an octahedral coordination compound having six ligands surrounding the metal atom/ion, we observe repulsion between the electrons in d orbitals and ligand electrons.
- This repulsion is experienced more in the case of dx2-y2 and dz2 orbitals as they point towards the axes along the direction of the ligand.
- Hence, they have higher energy than average energy in the spherical crystal field.
- On the other hand, dxy, dyz, and dxz orbitals experience lower repulsions as they are directed between the axes.
- Hence, these three orbitals have less energy than the average energy in the spherical crystal field.
Thus, the repulsions in octahedral coordination compound yield two energy levels:
- t2g– set of three orbitals (dxy, dyz and dxz) with lower energy
- eg – set of two orbitals (dx2-y2 and dz2) with higher energy
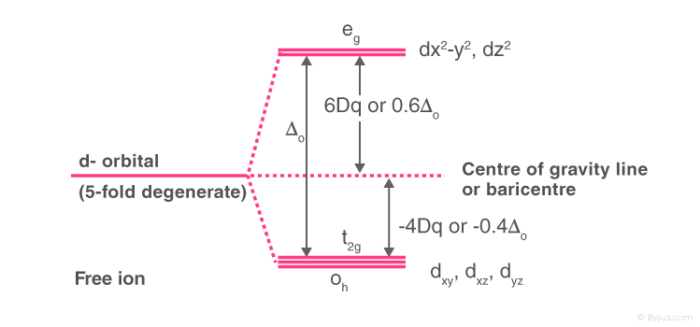
Crystal Field Splitting in Octahedral Complex
This splitting of degenerate level in the presence of ligand is known as crystal field splitting. The difference between the energy of t2g and eg level is denoted by “Δo” (subscript o stands for octahedral). Some ligands tend to produce strong fields thereby causing large crystal field splitting whereas some ligands tend to produce weak fields thereby causing small crystal field splitting.
Crystal Field Splitting in Tetrahedral Complex
The splitting of fivefold degenerate d orbitals of the metal ion into two levels in a tetrahedral crystal field is the representation of two sets of orbitals as Td. The electrons in dx2-y2 and dz2 orbitals are less repelled by the ligands than the electrons present in dxy, dyz, and dxz orbitals. As a result, the energy of dxy, dyz, and dxz orbital sets are raised while that of the dx2-y2 and dz2 orbitals are lowered.
- There are only four ligands in Td complexes and therefore the total negative charge of four ligands and hence the ligand field is less than that of six ligands.
- The direction of the orbitals does not coincide with the directions of the ligands approach to the metal ion.
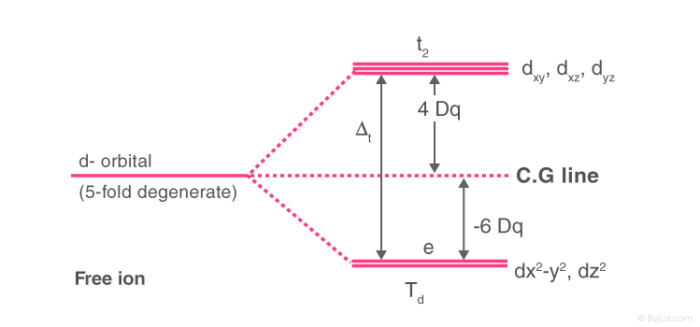
Crystal Field Splitting in Tetrahedral Complex
Thus, the repulsions in tetrahedral coordination compound yield two energy levels:
- t2– set of three orbitals (dxy, dyz and dxz) with higher energy
- e – set of two orbitals (dx2-y2 and dz2) with lower energy
The crystal field splitting in a tetrahedral complex is intrinsically smaller in an octahedral filed because there are only two thirds as many ligands and they have a less direct effect of the d orbitals. The relative stabilizing effect of e set will be -6Dq and the destabilizing effect of t2 set will be +4Dq
Crystal Field Stabilization Energy
In a chemical environment, the energy levels generally split as directed by the symmetry of the local field surrounding the metal ion. The energy difference between the eg and t2g levels is given as or 10Dq. It states that each electron that goes into the lower t2g level stabilizes the system by an amount of -4Dq and the electron that goes into eg level destabilizes the system by +6Dq. That is the t2g is lowered by 4Dq and the eg level is raised by +6Dq.
For example, the net change in energy for d5 and d10 systems will be zero as shown below.
d5 :- 3(-4Dq) + 2(+6Dq) = -12Dq + 12Dq = 0
d10 :- 6(-4Dq) + 4(+6Dq) = -24Dq + 24Dq = 0
The decrease in energy caused by the splitting of the energy levels is called the “Ligand Field Stabilization Energy (LFSE)”.
Crystal Field Stabilization Energy Table
The crystal field stabilization energies for some octahedral and tetrahedral complexes of 3d metal ions are tabulated below.
Electronic Configuration | Octahedral Complex | Tetrahedral Complex | ||
Weak Field (-Dq) | Strong Field (-Dq) | Weak Field (-Dq) | Strong Field (-Dq) | |
d0 | 0 | 0 | 0 | 0 |
d1 | 4 | 4 | 6 | 6 |
d2 | 8 | 8 | 12 | 12 |
d3 | 12 | 12 | 8 | (18)* |
d4 | 6 | 16 | 4 | (24)* |
d5 | 0 | 20 | 0 | (20)* |
d6 | 4 | 24 | 6 | (16)* |
d7 | 8 | 18 | 12 | 12 |
d8 | 12 | 12 | 8 | 8 |
d9 | 6 | 6 | 4 | 4 |
d10 | 0 | 0 | 0 | 0 |
Thus, the crystal field splitting depends on the field produced by the ligand and the charge on the metal ion. An experimentally determined series based on the absorption of light by coordination compound with different ligands known as spectrochemical series has been proposed. Spectrochemical series arranges ligands in order of their field strength as:
I– < Br– < Cl– < SCN– < F– < OH– < C2O42- < H2O < NCS– < EDTA4- < NH3 < en < CN–< CO
Filling of d-orbitals takes place in the following manner; the first three electrons are arranged in t2g level as per the Hund’s rule. The fourth electron can either enter into the t2g level giving a configuration of t2g4eg0 or can enter the eg orbital giving a configuration of t2g3eg1. This depends on two parameters magnitude of crystal field splitting, Δo and pairing energy, P. The possibilities of the two cases can better be explained as
- Δo > P – Electron enters in the t2g level giving a configuration of t2g4eg0. Ligands producing this configuration are known as strong field ligands and form low spin complexes.
- Δo < P – Electron enters in the eg level giving a configuration of t2g3eg1. Ligands producing this configuration are known as weak field ligands and form high spin complexes.
Frequently Asked Questions – FAQs
Who discovered crystal field theory?
Hans Bethe, a physicist, developed the crystal field theory (CFT) for crystalline solids in 1929.
What does crystal field theory explain?
Crystal field theory (CFT) describes the breaking of degeneracies of electron orbital states, typically d or f orbitals, caused by a static electric field generated by a surrounding charge distribution (anion neighbours).
What are the limitations of Crystal Field Theory?
Some limitations of CFT are as follows:
- This theory only considers the d-orbitals of a central atom. The s and p orbits are not taken into account in this study.
- The theory fails to explain the behaviour of certain metals, which exhibit large splitting while others exhibit minor splitting. For example, the theory provides no explanation for why H2O is a stronger ligand than OH–.
- The theory excludes the possibility of p bonding. This is a significant disadvantage because it is found in many complexes.
- The orbits of the ligands have no significance in the theory. As a result, it cannot explain any properties of ligand orbitals or their interactions with metal orbitals.
What are the advantages of Crystal Field Theory?
The following are some of the advantages of crystal field theory.
- This theory can be used to describe the stability of complexes. The greater the crystal field splitting energy, the greater the stability.
- Complexes’ colour and spectra can be explained using this theory.
- This theory explains complexes’ magnetic properties.
Why is CFT superior to VBT?
The mixing of orbitals during bond formation was explained by Valence Bond Theory (VBT). The explanation was primarily based on hybridisation concepts. While Crystal Field Theory explains how orbitals split as ligands approach metal ions, VBT was unable to adequately explain magnetic behaviour. It was unable to explain the formation of outer orbital and inner orbital complexes. CFT, on the other hand, explained everything.
Comments